Tracking indium at the Atom Tomography Probe at Curtin University
Over the past couple of weeks, I was immersed in an exhilarating adventure at the Atom Tomography Probe (ATP) located within the John de Laeter Research Centre at Curtin University in Perth, Western Australia. A team of brilliant minds, including Professor Steve Reddy, Dr David Saxey, Dr Denis Fougerouse, and Stefano Tenuta supported this scientific journey that holds immense promise.
ATP is a characterisation technique that combines high spatial resolution with mass spectrometry to provide 3-dimensional chemical information at the atomic scale. This experience forms an integral part of my ongoing PhD pursuit, where the focus is on uncovering the hidden secrets of a critical metal – indium – hosted in mine waste.
The goal? To foster a deeper knowledge of how this metal is resided to build a better understanding of its surficial cycling and contributing insights for potential recovery strategies.
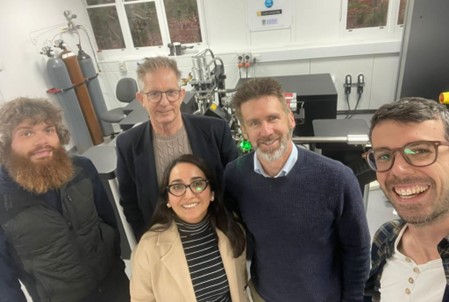
Embarking on ATP experiments is a complex journey, characterized by a series of meticulous preparatory steps and significant hurdles to overcome. The success of these experiments hinges on overcoming challenges in sample preparation and/or navigating the potential for specimens to rupture during analysis. Our first stride was to validate the crystallography of the targeted minerals – chalcopyrite, sphalerite, and stannite – chosen for their relevance in assessing indium distribution. Employing a technique called electron backscatter diffraction (EBSD), we mapped the crystal structures. These EBSD maps serve as invaluable guides for identifying microstructural complexities like grain boundaries, offering crucial insights for subsequent ATP targeting.
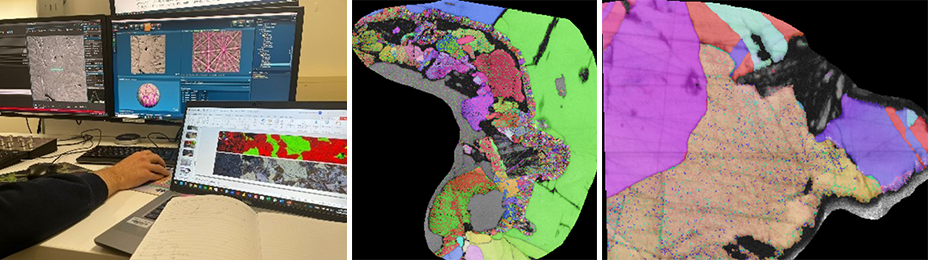
Second, we utilized a focused ion-beam scanning electron microscope (FIB-SEM) to prepare our atom probe specimens. This intricate process involved a series of steps: i) a rectangular platinum capping layer was meticulously deposited over the region of interest; ii) a triangular prism measuring 2.5 μm wide was delicately excised; iii) these prism segments were then meticulously milled to get a needle-like shape, culminating in a final tip diameter of under 100 nm.
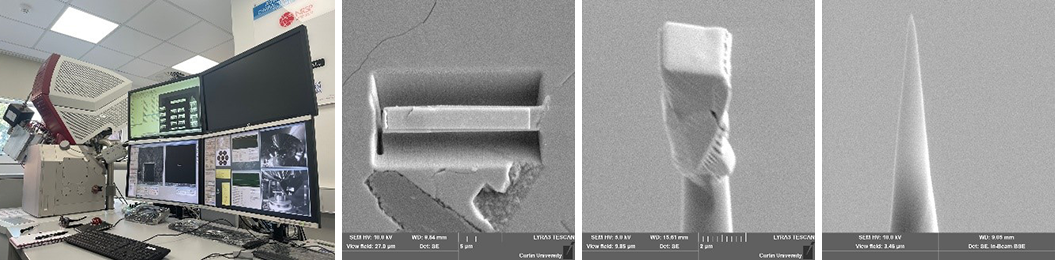
Third, these needles took center stage as we commenced the ATP process. The culmination of our efforts was nothing short of astounding – we captured stunning 3D images at nanoscale resolution for several needles including all the mineral phases of interest.
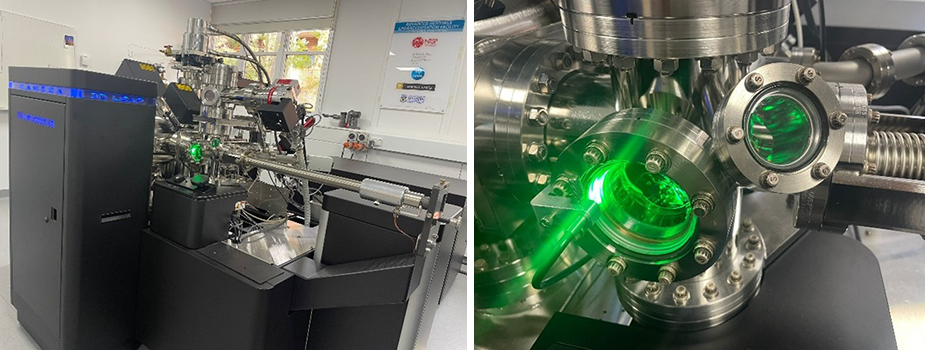
Through this lens, a captivating world unfolded, showcasing the indium's distribution not only in the crystal lattice of the targeted minerals, but also a truly heterogeneous manner at the nanoscale level. This intricate pattern of dispersion unveils a remarkable story of indium's affinity for segregating mainly with copper, and the presence of zinc, iron, silver, tin, and cadmium, within distinct mineral defects (grain boundary) and interfaces. The segregations have different shapes, as elongated features, C-like shape, clusters, and spherical forms. Some spheres are enriched in indium (up to 12% In). Here, we are potentially looking at the process of intermediate phases that are in process to exsolve an indium mineral phase. These defects and interfaces present energetically favourable havens for indium to establish residence. This holds profound implications, potentially influencing the efficiency of extraction processes.
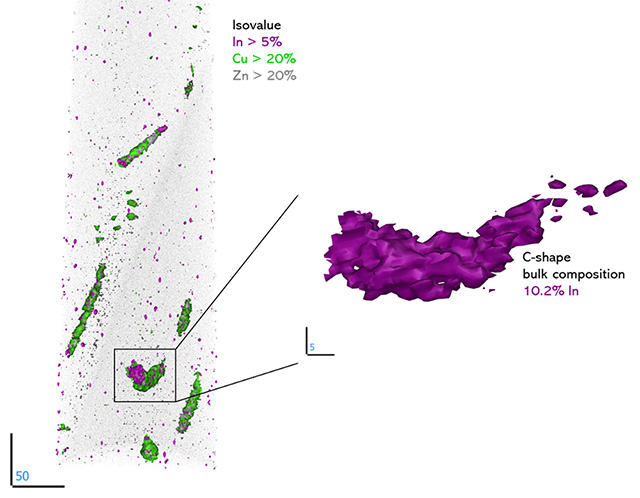
As an overall, my time at Curtin University has been nothing short of amazing. Looking ahead, delving deeply into the fresh data collected from the ATP experiments and integrating them with other techniques that have already shed light on the world of indium cycling within mine waste environments.
This research project was funded by Geoscience Atom Probe – AuScope Access Funding, and it is kindly supported by the Geological Survey of Queensland (GSQ) under the New Economy Minerals Initiative (NEMI) program.